Guardians of the Galaxy: Microgravity, Microbes, and Interplanetary Travel
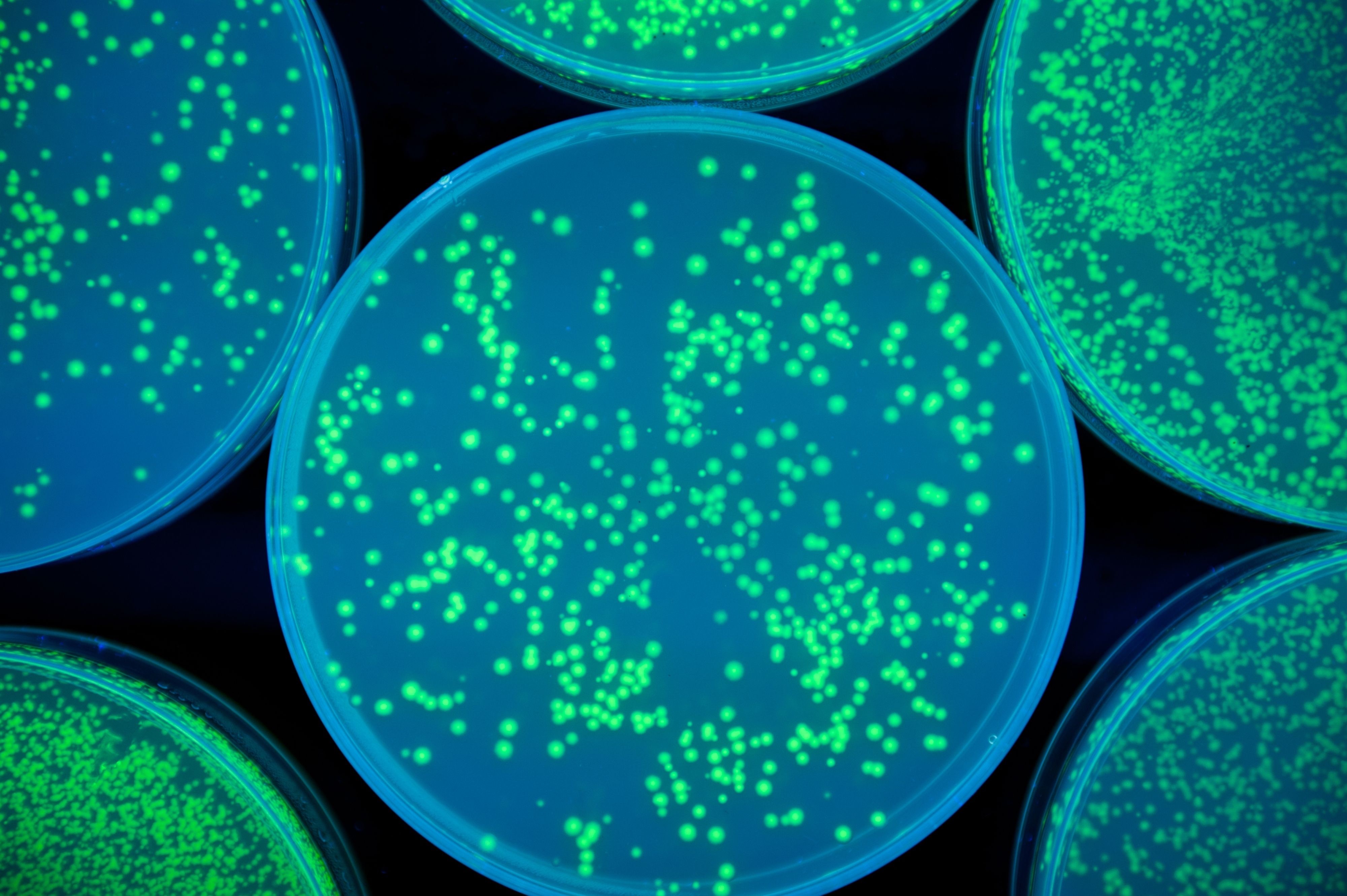
As humanity gears up for voyages to Mars and extended lunar vacations, predicting and managing microbial behavior is becoming a pressing priority. With longer missions on the table, understanding how microgravity affects bacteria is now a matter of life and death. From biomanufacturing to microbiomes, it is essential for the success of all our future ventures into space.
Yet conducting many experiments in space in a short span of time is prohibitively expensive.
Fortunately, simulating extreme environments is supercharging an array of research areas. Simulated microgravity is already yielding illuminating results in microbiology, thanks in part to Litegrav's high-throughput solutions.
Impact on Microbe Metabolism
Unlike on earth, where gravity dictates how fluids and nutrients move, the near-weightless environment of space forces microbes to respond in unexpected ways.
Primary metabolic pathways, those responsible for energy production and nutrient processing, are altered in space. E. coli exposed to microgravity tend to overexpress genes associated with glucose metabolism and thiamine synthesis—indicators of a stress response from limited nutrient availability (Liu, 2023).
Zea, et al. showed that E. Coli exposed to the same conditions as strains on earth showed a ten-fold overexpression of these genes linked to enhanced thiamine synthesis, crucial for glucose metabolism. It is thought that the starvation conditions of space lead to this augmentation (Zea, 2016). In truth, these germs often respond in opposite ways to uniform conditions (Sharma, 2022).
But microgravity does more than tweak primary metabolism; it can also cause a seismic shift in secondary metabolism—processes that produce antibiotics, pigments, and toxins (Huang, 2018). These secondary metabolites are critical for microbial survival in unforgiving conditions. Certain bacteria react with additional antioxidants and antibiotics for protection. Others may produce more toxins. This variability suggests that space conditions may contribute to virulence—antibiotic resistance or the increased production of harmful byproducts.
The administration of gentamicin to strains of E. coli on Earth and in space resulted in the space E. coli surviving at higher concentrations of the drug while thiamine gene expression was more robust than on earth (Aunins, 2018).
Even within the same species, metabolite production can be affected in many ways. Microgravity can also alter where and how these compounds are made (Sharma, 2022; Fang, 1997).
The spaceflight industry is seeing explosive growth in investments, expenditures, and launches. The Space Report for Q4 in 2023 heralded that year as the busiest on record. There were 223 launch attempts and 212 successful launches. Satellite launches rose by nearly a quarter and the entire commercial launch industry gained 50 percent in activity compared with the previous year (Space Foundation, 2024).
Long-Term Space Missions and Microbial Threats
Prolonged exposure to microgravity compromises our immune systems, leaving astronauts susceptible to infections. Studies on the International Space Station (ISS) have documented microbiome disturbances, including increases in skin bacteria associated with rashes and gut bacteria linked to inflammation (Green, 2021).
These changes are not merely uncomfortable; they could pose major risks on long missions where medical can be months or years away. If a microbe were to develop enhanced virulence or antibiotic resistance, it could lead to infections that are impossible to treat with the limited medical supplies available on a spacecraft.
Harnessing Microbes for Space Exploration
Biotechnology is letting us harness microorganisms for a range of applications, from bioremediation—cleaning up waste products on spacecraft—to biomanufacturing, where engineered bacteria make vital nutrients, medicines, or even oxygen. Using microbes to maintain closed-loop life support systems is particularly exciting for long-term missions, as resupplying would be impractical or impossible.
Experiments are already underway to engineer microbes that can thrive in space and perform useful tasks. Bacteria modified to produce antibiotics or vitamins could be cultured aboard spacecraft, reducing the need for medical supplies.
Microbial Evolution in Space: A New Frontier
One of the most intriguing questions in microbiology is how life adapts to hostile environments over many generations. Alongside changes in gene expression, microgravity and radiation could accelerate microbial evolution, leading to the emergence of new (and potentially undesirable) traits. Some microbes exhibit hastened mutation rates in space, potentially giving rise to new, more resilient strains (Weng, 1999).
Moreover, gravity’s absence could facilitate horizontal gene transfer between microbes, rapidly spreading advantageous genes (which, from our perspective, may not always be a good thing). Understanding these processes could help us manage microbial risks on earth while delivering insights into the fundamental principles of life in extreme environments (Urbaniak, 2021).
Conclusion
The study of microbes in microgravity is more than just an exploration of life at the microscopic scale. It’s a burgeoning and fascinating field that holds the key to the success of future space missions and the health of astronauts. As we prepare to venture deeper into space, understanding how these tiny organisms adapt, survive, and thrive in unusual conditions will be essential.
Doing this in a cost-effective manner will require technologies like those provided by Litegrav. From ensuring the safety of space crews to leveraging microbial biotechnology, the research conducted today will pave the way for the space exploration of tomorrow.
References and Suggested Reading
Institute of Medicine (US) Committee on Creating a Vision for Space Medicine During Travel Beyond Earth Orbit; Ball JR, Evans CH Jr., editors. Safe Passage: Astronaut Care for Exploration Missions. Washington (DC): National Academies Press (US); 2001. 2, Risks to Astronaut Health During Space Travel. Available from: https://www.ncbi.nlm.nih.gov/books/NBK223785/.
McLean RJC, Cassanto JM, Barnes MB, Koo JH. Bacterial Biofilm Formation under Microgravity Conditions. FEMS Microbiol Lett. 2001;195:115–119. doi: 10.1111/j.1574-6968.2001.tb10507.x.
Milojevic T, Weckwerth W. Molecular Mechanisms of Microbial Survivability in Outer Space: A Systems Biology Approach. Front Microbiol. 2020 May 15;11:923. doi: 10.3389/fmicb.2020.00923. PMID: 32499769; PMCID: PMC7242639.
Mora M, Wink L, Kögler I, Mahnert A, Rettberg P, Schwendner P, Demets R, Cockell C, Alekhova T, Klingl A, Krause R, Zolotariof A, Alexandrova A, Moissl-Eichinger C. Space Station conditions are selective but do not alter microbial characteristics relevant to human health. Nat Commun. 2019 Sep 5;10(1):3990. doi: 10.1038/s41467-019-11682-z. PMID: 31488812; PMCID: PMC6728350.
Nickerson CA, Ott CM, Wilson JW, Ramamurthy R, Pierson DL. Microbial responses to microgravity and other low-shear environments. Microbiol Mol Biol Rev. 2004 Jun;68(2):345-61. doi: 10.1128/MMBR.68.2.345-361.2004. PMID: 15187188; PMCID: PMC419922.
Rampelotto PH. Extremophiles and extreme environments. Life (Basel). 2013 Aug 7;3(3):482-5. doi: 10.3390/life3030482. PMID: 25369817; PMCID: PMC4187170.
Santamaria G, Liao C, Lindberg C, Chen Y, Wang Z, Rhee K, Pinto FR, Yan J, Xavier JB. Evolution and regulation of microbial secondary metabolism. Elife. 2022 Nov 21;11
. doi: 10.7554/eLife.76119. PMID: 36409069; PMCID: PMC9708071.
Scribani Rossi C, Barrientos-Moreno L, Paone A, Cutruzzolà F, Paiardini A, Espinosa-Urgel M, Rinaldo S. Nutrient Sensing and Biofilm Modulation: The Example of L-arginine in Pseudomonas. Int J Mol Sci. 2022 Apr 15;23(8):4386. doi: 10.3390/ijms23084386. PMID: 35457206; PMCID: PMC9028604.
Sharma G, Curtis PD. The Impacts of Microgravity on Bacterial Metabolism. Life (Basel). 2022 May 24;12(6):774. doi: 10.3390/life12060774. PMID: 35743807; PMCID: PMC9225508.
Singavarapu B, Du J, Beugnon R, Cesarz S, Eisenhauer N, Xue K, Wang Y, Bruelheide H, Wubet T. Functional Potential of Soil Microbial Communities and Their Subcommunities Varies with Tree Mycorrhizal Type and Tree Diversity. Microbiol Spectr. 2023 Apr 13;11(2)
. doi: 10.1128/spectrum.04578-22. PMID: 36951585; PMCID: PMC10111882.
Taylor PW. Impact of space flight on bacterial virulence and antibiotic susceptibility. Infect Drug Resist. 2015 Jul 30;8:249-62. doi: 10.2147/IDR.S67275. PMID: 26251622; PMCID: PMC4524529.
Zea L, Prasad N, Levy SE, Stodieck L, Jones A, Shrestha S, Klaus D. A Molecular Genetic Basis Explaining Altered Bacterial Behavior in Space. PLoS One. 2016 Nov 2;11(11)
. doi: 10.1371/journal.pone.0164359. PMID: